The Pseudo-Hyperbolic Metric and Lindelöf's Inequality (cont.)
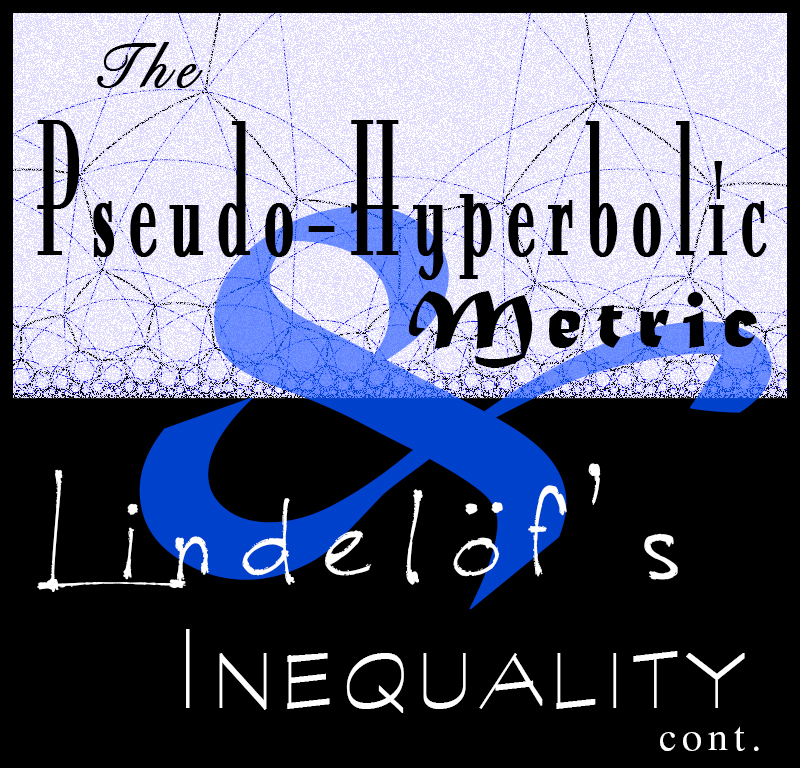
Last time, we proved that the pseudo-hyperbolic metric on the unit disc $\Delta\subset \mathbb{C}$ given by $$d(z,a)=|\varphi_a(z)|=\bigg|\frac{z-a}{1-\bar a z}\bigg|$$ is indeed a metric. As an application, our next goal is to verify the following inequality due to Lindelöf.
Theorem (Lindelöf's Inequality). Every holomorphic function $f:\Delta\to\Delta$ satisfies $$\frac{|f(0)|-|z|}{1-|f(0)||z|}\leq |f(z)|\leq \frac{|f(0)|+|z|}{1+|f(0)||z|}\quad\text{for all $z\in\Delta$}.$$
Notice that if $f(0)=0$, then the above reduces to the same bound on $|f(z)|$ that we get from the Schwarz Lemma. So in effect, Lindelöf's inequality says, "If you don't know that $f(0)=0$, here's what you do know." (Fun fact: In 1938, Lars Ahlfors proved* that the Schwarz Lemma is actually a statement about curvature of Riemannian metrics! For more on this, see, for instance, ch. 2 of Krantz's Geometric Function Theory.) Now to prove the theorem, we first need to derive a useful inequality concerning the metric $d$.
Claim. For all $z,a\in \Delta$, the metric $d$ satisfies $$d(|z|,|a|)\leq d(z,a)\leq d(|z|,-|a|).$$
Proof. We need only prove the first inequality since the second was verified during the proof of the proposition in our previous post. To this end, note that $$d(|z|,|a|)=\bigg| \frac{|z|-|a|}{1-|z||a|} \bigg| = \frac{| |z|-|a| |}{1-|z||a|} $$
and so \begin{align}\label{there} 1-d(|z|,|a|)^2 &= \frac{(1-|z|^2)(1-|a|^2)}{(1-|z||a|)^2}. \end{align} But the above is less than or equal to $1-d(z,a)^2$. Indeed, consider the following: $$1-d(z,a)^2=\frac{(1-|z|^2)(1-|a|^2)}{|1-\bar a z|^2} \leq \frac{(1-|z|^2)(1-|a|^2)}{(1-|z||a|)^2}=1-d(|z|,|a|)^2 .$$ The leftmost equality was derived in the proof of the proposition, and the inequality follows since $|1-\bar a z|\geq ||1| - |a\bar z||= |1- |a| |z||$. Of course the rightmost equality is (\ref{there}). From this we conclude $d(|z|,|a|)\leq d(z,a)$ as desired.
We are now ready to derive Lindelöf's inequality and do so by verifying the rightmost inequality first. Again let $z\in\Delta$. By our claim above we know that $$d(|f(z)|,|f(0)|)\; \leq \;d(f(z),f(0))\; \leq \; d(|z|,0)$$ which by definition of $d$ implies \begin{align}\label{blue} \bigg| \frac{|f(z)|-|f(0)|}{1-|f(z)||f(0)|} \bigg| \leq |z| \end{align} and so $||f(z)|-|f(0)||\leq |z|(1-|f(z)||f(0)|)$. Thus \begin{align*} |f(z)|&\leq ||f(z)|-|f(0|| + |f(0)| \\ &\leq |z| (1-|f(z)||f(0)|) + |f(0)|. \end{align*} Rearranging terms we find that $|f(z)|( 1 + |z||f(0)|)\leq |f(0)| + |z|$ and so $$|f(z)|\leq \frac{|f(0)|+|z|}{1+|f(0)||z|}$$ as desired. For the lower bound on $|f(z)|$ observe that (\ref{blue}) implies \begin{align*} |f(0)|&\leq ||f(0)|-|f(z)||+|f(z)|\\ &\leq |z|(1-|f(z)||f(0)|)+|f(z)| \end{align*} and hence, again with some rearranging, $|f(0)|+|z||f(z)||f(0)|\leq|f(z)|+|z|$ and thus $$\frac{|f(0)|-|z|}{1-|f(0)||z|}\leq |f(z)|.$$
QED!
*L. Ahlfors, An extension of Schwarz’s lemma, Trans. Amer. Math. Soc. 43 (1938), 359–364.