Automorphisms of the Upper Half Plane
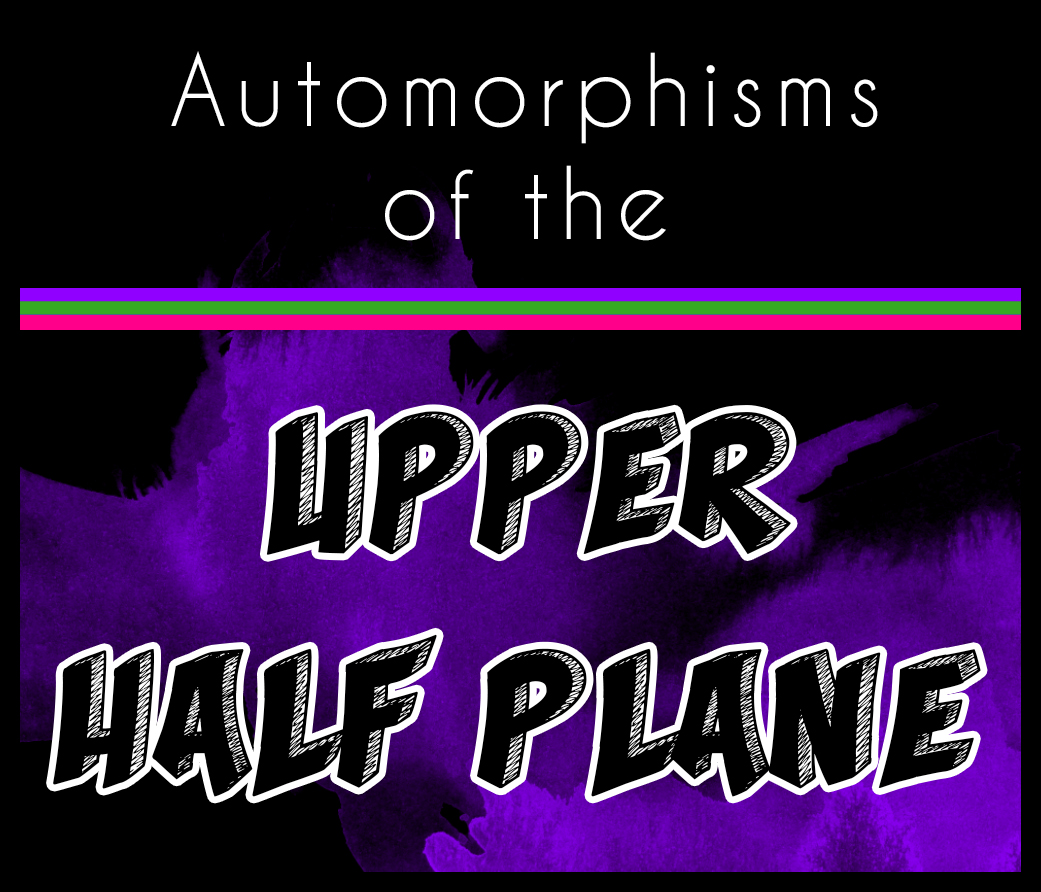
Welcome back to our little series on automorphisms of four (though, for all practical purposes, it's really three) different Riemann surfaces: the unit disc, the upper half plane, the complex plane, and the Riemann sphere. Last time, we proved that the automorphisms of the unit disc take on a certain form. Today, our goal is to prove a similar result about automorphisms of the upper half plane.
If you missed the introductory/motivational post for this series, be sure to check it out here!
Also in this series:
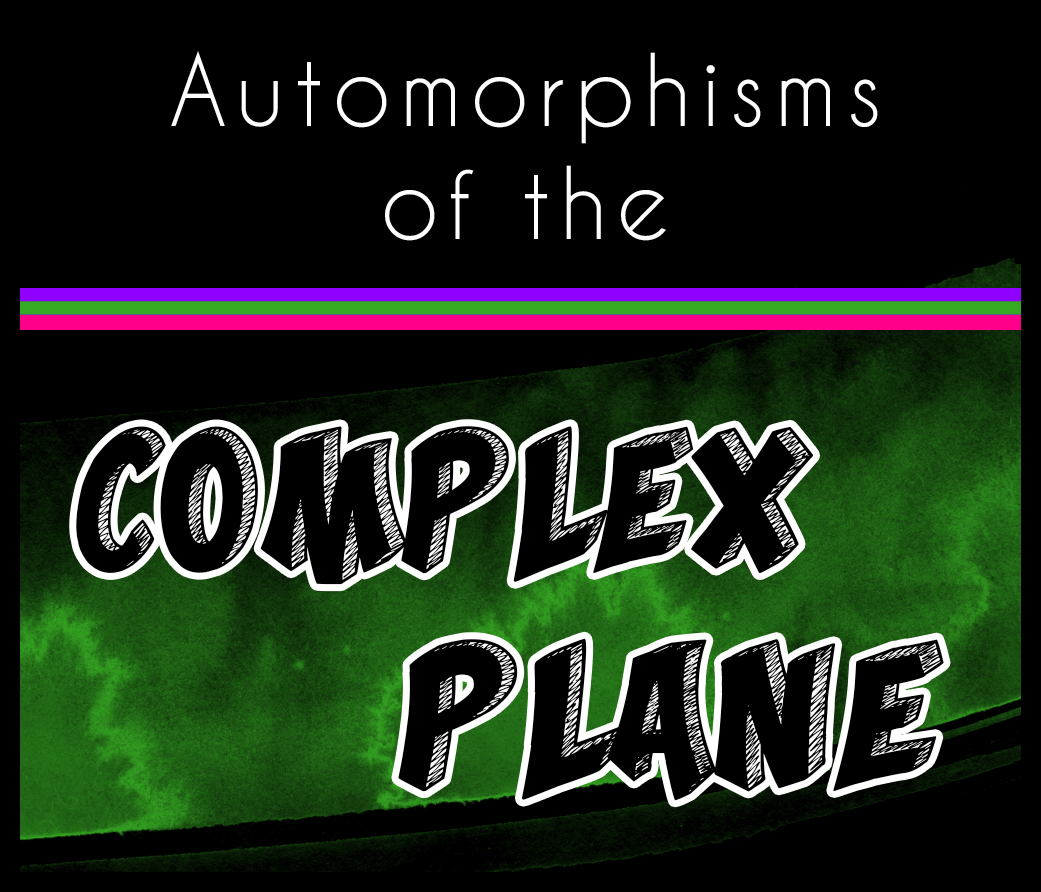

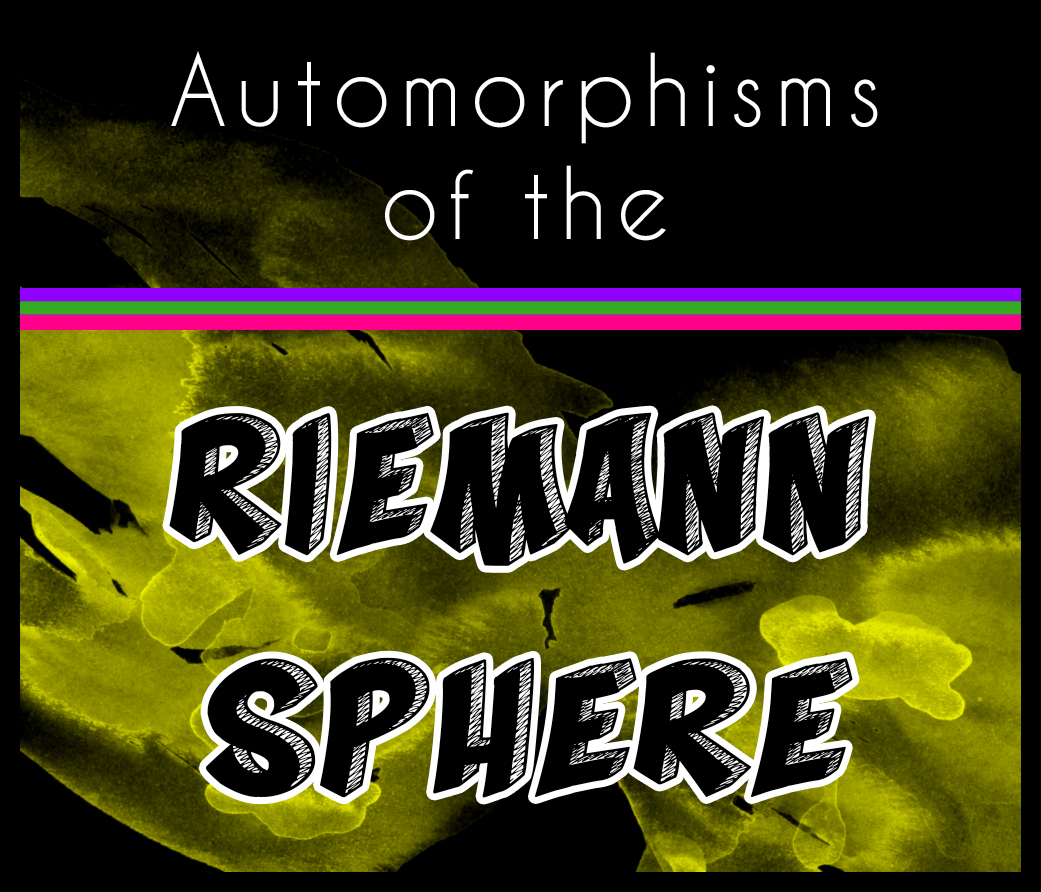
Automorphisms of the Upper Half Plane
Theorem: Every automorphism $f$ of the upper half plane $\mathcal{U}$ is of the form $f(z)=\frac{az+b}{c z+d}$ where $a,b,c,d\in\mathbb{R}$ and $ad-bc=1$.
Proof. First let's suppose $f\in\text{Aut}(\mathcal{U})$ and recall (or observe) that the function $g:\mathcal{U}\to\Delta$ given by $$g(z)=\frac{z-i}{z+i}$$ is a conformal map. (Here, $\Delta$ denotes the unit disc.) Define $F=g\circ f\circ g^{-1}:\Delta\to\Delta$ so that $F\in\text{Aut}(\Delta)$, that is, $F$ is an automorphism of $\Delta$. By our previous post, there exists $a,b\in\mathbb{C}$ with $|a|^2-|b|^2=1$ such that $$F(t)=\frac{at+b}{\bar bt + \bar a}, \qquad t\in\Delta.$$ Now let $z=g^{-1}(t)\in\mathcal{U}$ so that $g(z)=t$. Then since $f=g^{-1}\circ F \circ g$ and $g^{-1}(w)=\frac{i+wi}{1-w}$ for any $w\in\Delta$ we have $$f(z)=g^{-1}(F(g(z)))=g^{-1}\left( \frac{ a\left(\frac{z-i}{z+i} \right) + b }{\bar b\left(\frac{z-i}{z+i} \right) + \bar a } \right)$$ which reduces to \begin{align} \frac{z(ai+bi+\bar a i +\bar bi) -\bar a +\bar b - b +a }{z(\bar a +\bar b - a - b) +\bar ai-\bar bi-bi+ai }. \end{align} Since $a=x+iy$ and $b=p+iq$ for real numbers $x,y,p,q$, we see that \begin{align*} ai=-yix \qquad&\text{and}\qquad \bar ai=y+ix,\\ bi=-q+ip \qquad&\text{and}\qquad \bar bi=q+ip,\\ \bar a - a = -2iy \qquad&\text{and}\qquad \bar b - b=-2iq. \end{align*} Thus (1) becomes $$\frac{z(x+p) + (y-q)}{z(-y-q)+(x-p)}$$ which is of the form $\frac{Az+B}{Cz+D}$ where $A,B,C,D\in\mathbb{R}$ and $AD-BC=(x^2-p^2)+(y^2-q^2)=(x^2+y^2)-(p^2+q^2)=|a|^2-|b|^2=1$ as desired.
Conversely suppose $f(z)=\frac{az+b}{cz+d}$ for $a,b,c,d\in\mathbb{R}$ where $ad-bc=1$ for $z\in\mathcal{U}$. To show $f\in\text{Aut}(\mathcal{U})$, the only potentially tricky part is to show that $f(z)\in\mathcal{U}$, i.e. $\text{Im}f(z)>0$. (Note that $f$ is a linear fractional transformation, so it's holomorphic and invertible and it's inverse is also holomorphic.) But in fact it's not hard at all! Simply notice that if $z=x+iy$ then \begin{align*} f(z)&=\frac{(az+b)(c\bar z+d)}{|cz+d|^2}\\ &=\frac{ac(x^2+y^2) +bc(x-iy) + ad(x+iy) + bd }{|cz+d|^2} \end{align*} and so Im$f(z)=\frac{ad-bc}{|cz+d|^2}y=\frac{\text{Im}(z)}{|cz+d|^2}$ and this is strictly greater than zero since $\text{Im}(z)>0$.
$\square$
Next time: We'll prove a similar result about the automorphisms of the complex plane.